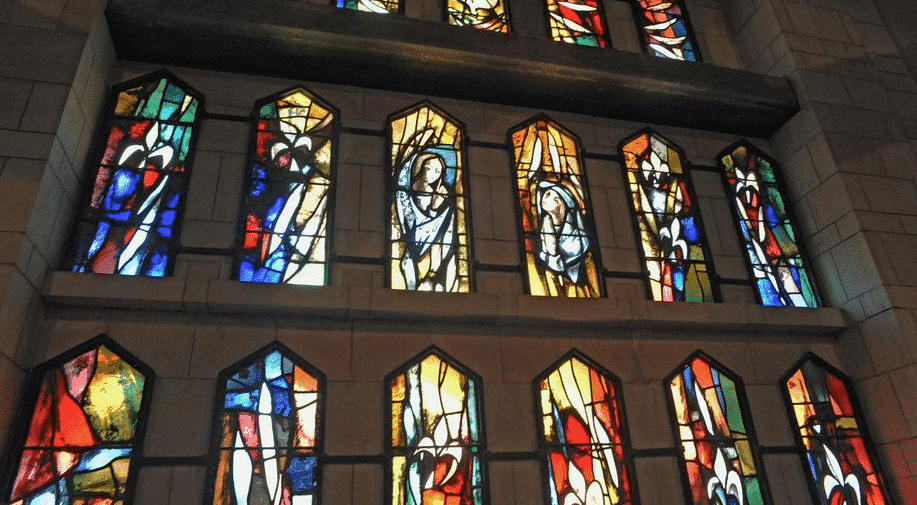
Abstract
Stained-glass windows form an important part of cultural heritage. Short pulse lasers open new opportunities for safe decontamination. In this work, and for the first time, the interaction of sub-nanosecond and femtosecond pulsed lasers with some contemporary stained-glasses has been analyzed exploring their applicability to safely clean stained-glass windows. The results show that, in these materials, damage can be induced using energy levels below damage thresholds due to the heat accumulation in the coating layer that is being eliminated. The latter generates significant thermal stresses on the glass volume, which induce crack formation. In consequence, in order to apply safe stained-glass cleaning protocols, laser parameters have to be selected to control the temperature increase within this layer. To achieve the latter goal, two alternative irradiation procedures were explored in this work. As a first option, a reduction of the effective pulse repetition frequency to values lower than 20 kHz was applied. A second alternative was used for lasers with pulse repetition rates in the hundreds of kHz. In this case, the burst mode was employed controlling the number of pulses emitted and combined with an adequate time lapse selection between two consecutive burst runs. A proof of concept demonstration was carried out on a stained-glass sample from the Cathedral of Cuenca, dated at the end of the XV century.
Materials and methods – Stained-glass samples
Four contemporary stained-glass samples of different colours (green, colourless, red and blue) were selected for this study. These samples were supplied by the glass factory of Verreire de Saint-Just, located in Saint-Just-Saint-Rambert, in Loire (France). This factory was founded about 189 years ago. Currently, Verreire de Saint-Just glass maker is a reference supplier of glass and stained-glass in Europe. The samples have a rectangular shape with dimensions 4 cm × 1.5 cm × 2 mm. Elemental chemical compositions were determined by EDS analyses and are presented in. The major components found in the green, colourless and blue glasses include SiO2, Na2O, CaO, and MgO. Differences in colours are reflected in the presence of small amounts of other elements, as for instance Cr and Cu in the green glass. The red glass differs from the above. In this case, the glass exhibits an external layer of 267 μm with a different composition. Its bulk composition is similar to that of the other glasses, but its external layer includes SiO2, Na2O and K2O as main components, as well as important amounts of Zn and Ba.
Optical and surface characterization
The differences in colour between the glasses suggest that they can exhibit different absorption properties at the emission wavelength of the laser. The transmittance and absorption values in the UV–Vis–nIR range, in particular between 300 and 1100 nm, were characterized using a StellarNet Miniature Spectrometer. A SL1-CAL light source, 0.6 mm in diameter and a cone angle of 25.4° at the output of the optical fibre and specifically configured for irradiance calibrations in the 300–1100 nm range, was used. Transmittance tests were carried out with an integration time of 5 ms, averaging 100 scans.
Analysis of optical properties
The transmittance spectra above corresponds to the different types of contemporary glasses studied. For each sample, spectra were acquired in three different positions, in order to obtain information about the homogeneity of the optical response in each glass. In all the cases, differences lower than 10% are only observed in the regions of the spectra with transmittance values higher than a 70%. These differences have been associated to the distribution of micro-bubbles inside the glass in the illuminated (approximately 1 cm in diameter) areas.
As expected, the differences in colours generate very different transmittance spectra. For example, in the spectral range close to 1064 nm colourless, red and blue glasses absorb less than 20%. The amount of energy that is absorbed by the green glass, however, increases to values approaching 70%. In the region of the spectrum close to 530 nm, corresponding to the emission of green lasers, it is also observed that the amount of absorbed energy is very high in the three coloured glasses and only the colourless one presents high transmittance values. Finally, the four glasses, in particular the green and red one, exhibit lower transmittance values in the proximity of 355 nm, a characteristic emission wavelength for third harmonic (UV) solid state and rare-earth fibre lasers.
Conclusions
This work demonstrates that when IR laser cleaning is applied to remove contaminating layers from the surface of a glass, the limiting factor is the control of the maximum temperature reached within the outermost layer, even if the levels of the applied laser irradiance or fluence values are lower than those specified for the glass damage threshold. Several experiments have shown that laser treatments that do not cause any direct damage to the glass, deteriorate it if they are applied to remove contaminants that are deposited on the glass surface. The heat generated on the contaminant material to be cleaned induces a level of thermal stress which is high enough to cause mechanical cracks on the glass. In consequence, one of the main objectives of an ideal set of laser parameters to establish an effective cleaning protocol is to minimize this heat input, referred in this case as heat accumulation.
When high frequency burst laser treatments are applied, the temperature reached is limited by selecting a maximum number of pulses at each position of the burst series and introducing a time lapse between each series. In the particular case of the n-IR 800 ps laser used in this work, pulses of 24 μJ (Fluence 0.49 J/cm2 and Irradiance 0.61 GW/cm2) in treatments with 200 pulses for 10 cycles or more, with non-irradiation time-lapse intervals between each treatment, resulted in a more observable and effective cleaning. This was also proved to be effective at avoiding micro-cracks and melting in the substrate, even if the glass shows an important level of absorption.
On the other hand, some new lasers have the possibility of reducing effective pulse repetition frequency while maintaining the energy per pulse. In this case, the reduction of such frequency is sufficient to define safe cleaning protocols, even if scanning speeds are also reduced to maintain a desired pulse overlap. This was demonstrated in the present work using a fs laser-based treatment which provided irradiance values in the proximity of 104 GW/cm2. Safe laser treatments have thus been achieved limiting the pulse repetition frequency to 20 kHz or below.
These ideas are also essential in designing laser protocols that avoid glass damage in historic stained-glass windows. Once damage thresholds have been established for a given stained-glass, the laser cleaning protocol has to be defined limiting the temperature rise during the irradiation treatment, in order to avoid deterioration of the glass as a consequence of thermal shock.